
The overarching theme of the Yang group’s research is to combine atomic-level synthesis with femtosecond control, and hence tuning quantum materials into new territories where the fundamental many-body phases will be modified. In this process we will also discover new materials and new physics enabling applications in spintronics, topotronics, and quantum information sciences.
Spatial and temporal engineering of FeSeTe topological superconductors
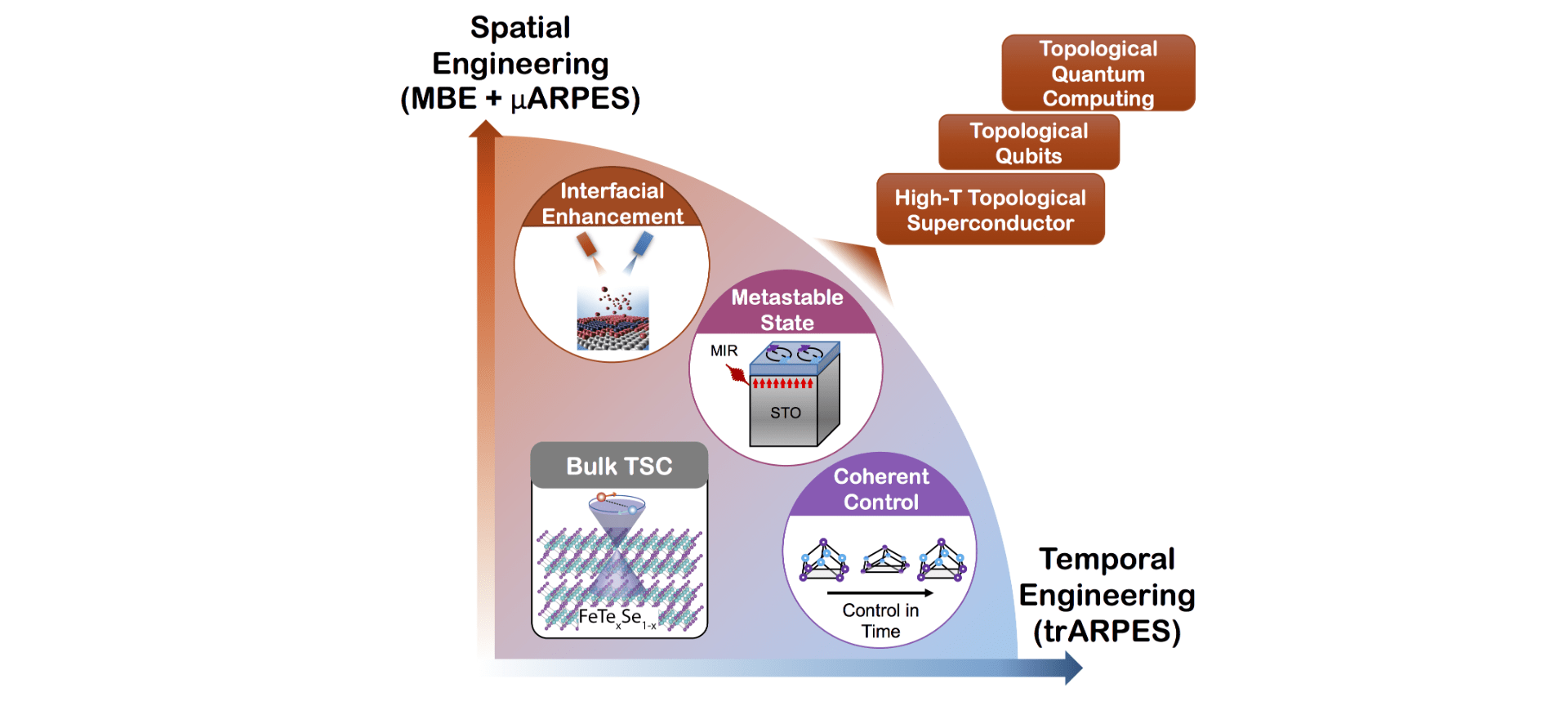
Topological superconductors (TSCs) are promising new platforms for realizing topological quantum computing. Compared to other material platforms such as Josephson junctions, cold atom systems, rare earth ions, and color centers in diamonds, topological quantum computing uses the topologically protected quantum states – so-called Majorana zero modes – to perform computing tasks. Theoretically, topological quantum computers are immune to both classical and quantum errors, potentially circumventing one of the biggest challenges in building a scalable quantum computer – the quantum error correction. It is thus of imminent interest to quantum scientists to explore the possibility of realizing topological quantum computing on solid state systems. The key is to be able to fabricate and manipulate TSCs.
We will use our newly established Microscopy And Spectroscopy for Transient Electronic-matter Research (MASTER) Platform to systematically control and characterize FeSeTe thin films as candidates for intrinsic high temperature TSCs. We will look into three directions: 1) programmable construction of TSCs and Majorana modes using oxide-chalcogenide superlattices; 2) light-induced metastable high temperature TSCs; 3) coherent control of topological phase transitions. These studies will not only provide novel insight into how to construct and control TSCs, but also enable us to engineer these materials for potential realizations of topological quantum computers.
Spatial and temporal engineering of magnetic topological insulators

The concept of topology has changed our perspectives on how to understand basic material properties. It allows us to predict and realize topologically protected edge or surface electronic states, the nature of which is free from material details. This leads to peculiar quantum transport behaviors, such as the Quantum Spin Hall Effect and Quantum Anomalous Hall Effect. Understanding how to precisely manipulate topological phases, particularly above the liquid helium temperatures, will lay the foundation for novel device concepts such as the topotronics.
Magnetic topological insulators are materials where intrinsic magnetism couples strongly to the topological electronic states, giving rise to a versatile material platform where distinct topological phases can be realized and tuned. We will use MRSTEP to manipulate the nonequilibrium topological phases of magnetic topological insulators, specifically the (MnBi2Te4)m(Bi2Te3)n family. We will fabricate (MnBi2Te4)m(Bi2Te3)n superlattices with atomic precision via molecular beam epitaxy, and disentangle spectroscopic features corresponding to different layer thicknesses and terminations via μARPES. We will also employ time-resolved ARPES to induce nonequilibrium topological changes of the electronic structure. Ultimately, the combination of precise spatial control and nonequilibrium engineering can lead to the revelation and manipulation of a nonequilibrium quantum anomalous Hall insulator state.
Miniaturized molecular beam epitaxy

One fundamental technology defining the modern society is the fabrication of nanoscale electronic devices. This technology has been traditionally separated into two steps: synthesis of high-quality wafer-scale thin films and nanofabrication enabled by photo- or electron-beam lithography. As the era of quantum engineering emerges, scientists and engineers are designing, building, and testing new information processing architectures at an ever-faster pace, and often need exquisite control over microscopic structures or devices. In this project, we will develop a novel synthesis technique based on a scanning probe platform – a miniaturized molecular beam epitaxy system. The scanning probe will be customized to serve as a deposition shadow mask for molecular beams, and enable deposition at sub-micron spatial scale. If successful, this new platform will integrate material synthesis and nanofabrication in one step, and allow a programmable printing of solid-state quantum circuits. The applications include (1) 2D superconducting devices based on iron selenide monolayer films; (2) nano-islands of oxide materials hosting individual to few-body rare-earth ions as entangled qubits; (3) using atomic sensors to probe the nanoscale magnetic and electronic domains in quantum materials; (4) hybrid quantum sensors composed of rare-earth oxide islands and nitrogen-vacancy centers.
NSF Award#2019131
Cavity quantum electrodynamics enhanced many-body phases
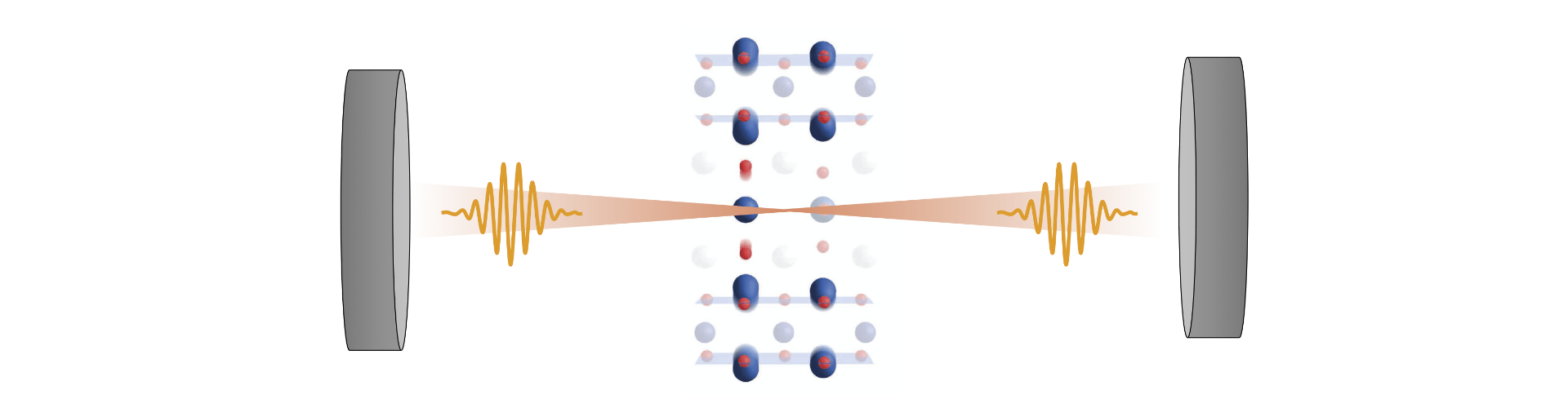
A striking manifestation of quantum mechanics is that light can profoundly modify the quantum states of matter, and even become inseparable with matter through strong coupling. The entangled states of light with atoms, defects, and molecules have been characterized with great precisions. However, despite the excitement and many tantalizing theoretical proposals, to this day it remains challenging to couple light in a similar fashion with the quantum many-body phases in solid-state systems which involve more than 10²³ electrons. If this challenge is overcome, it will lead to an unprecedented avenue to quantum mechanically control material properties for both fundamental scientific inquiries and future applications. A particularly exciting possibility is to access and stabilize nonequilibrium material phases which do not exist in nature. We will create and control strongly coupled quantum states involving light, electrons, and lattice vibrations, which may subsequently drive a substantial change in topological states and superconducting states. In this project we will leverage the frontier nanofabrication techniques to confine light in a micron-scale region, and substantially enhance the light-matter interaction. This effort will be based on the success of the Yang lab integrating a state-of-the-art thin film deposition system and an in situ microscopic electron spectroscopy setup. Moreover, the concept can be generalized to creating an array of phase coherent entangled states, providing a platform for quantum transduction and quantum networks.